Remembrance of Things Past:
Greenhouse Lessons
from the Geologic Record
by
Thomas J. Crowley
Global climate--like local weather--is ever changing, on all time scales, in response to natural variations. For this reason, projections of global warming, resulting from increasing levels of atmospheric greenhouse gases, need to be set in the context of what has happened in the past. A knowledge of what has changed, and when and where, provides insights into why past changes have occurred. Such information can also help in evaluating the reliability of global warming forecasts.
Predictions of climate change are based on numerical models of the atmosphere and ocean. The inherent limitations in these models have led those who develop and refine them to seek ways in which projections can be tested and sharpened, including a comparison with times when conditions on the Earth were much different from the present day. We should like to know, for example, how concentrations of greenhouse gases--or other factors that fix the climate of the Earth--have varied in the past. How has surface temperature responded? Is there evidence for natural controls that limit the range through which climate naturally varies?
To answer these and other questions, geologists and climatologists have literally unearthed a vast amount of information regarding climate and how it has varied. Natural records that tell of past changes are scattered over the globe. They can be recovered only piece by piece, and reassembled like a jigsaw puzzle, through the efforts of many people over many years. What emerges is not only a record of climate change, but a clearer picture of the climate system itself.
Can we hope to find in the past a time when temperatures rose by the amount that some models now project for next 100 years, to ascertain how the natural world reacts to such conditions? As we shall see, although the Earth has seen times as warm as are now projected, there are no exact parallels. But a great deal can be learned by examining what we know of times when conditions were something like what we now might expect, and even more from a knowledge of how the climate system has responded to induced changes of any sort.
In this review we look at some of the findings that allow us to place modeled projections of greenhouse warming in the context of past, or paleoclimates. In doing so we take as a baseline for comparison the climate of the present era and the range of global surface temperature increase that has been projected by the most recent assessment of the Intergovernmental Panel on Climate Change (IPCC): a "mid-range" rise of about 1 to 3° Centigrade (about 2 to 5° Fahrenheit) for the end of the next century.
First, we review what is known of how the climate has varied in the past, and the sources from which this information is obtained. We then cite some of the lessons that can be drawn from a closer look at the paleo record.
SOURCES OF CLIMATE HISTORY
Records of local weather, made with the help of meteorological instruments, cover at most about two hundred years, and an even shorter span if a truly global picture is desired. Other, historical accounts exist for individual places--most notably in China, where for certain sites they extend back two thousand years. But although these records are useful, more extensive information is required to understand the full range of natural climate variability.
Laboratory analyses of geologic sediments and other layered materials help meet this need, extending what is known of surface temperature, precipitation, and other meteorological parameters many thousands and even millions of years into the past. Many of these tools rely on the fact that plants and other forms of life respond in distinctive ways to changes in the local environment, thus preserving an indirect, or proxy record, of climatic conditions.
Annual growth rings in trees, for example, can be read much like a diary: tree-ring widths tell of seasonal variations of local air and water conditions--as does the chemical composition of the wood within each ring. The presence of forests and other vegetation, which are indicators of climatic conditions, can be reconstructed from the analysis of pollen in lake sediments. Cores extracted from the floor of the ocean allow us to examine in fossil form the microscopic life that once lived near the ocean surface, and through this analysis, to recover information about the temperature of the ocean many millions of years ago. The extent and composition of coral reefs are indicators of tropical ocean temperatures and, through changes in ocean salinity, of local precipitation.
A particularly powerful technique of recent years has been the recovery and analysis of ice cores, about ten cm (four inches) in diameter and as much as two miles long, drawn from the permanent glaciers on Greenland and Antarctica. Similar samples have been retrieved from high mountain glaciers in South America and Asia. As in the case of trees, the ice is composed of annual layers, although the temporal resolution degrades systematically from top to bottom in the deepest cores.
The analysis of the hydrogen and oxygen in the extracted ice core provides a continuous index of temperature from as far back as 200,000 years ago, sampling conditions in the air above the ice sheet and in the nearby oceans from which the water was evaporated, to later fall as snow. As the snow accumulates, over the years, the underlying layers are compressed into ice. Windblown dust and the residue of ancient volcanic eruptions can also be analyzed. Bubbles entrapped in the ice during the process of compaction preserve samples of fossil air, affording an opportunity for precise measurement of the amount of carbon dioxide (CO2), methane (CH4), and other greenhouse gases in the global atmosphere of long ago.
These techniques are calibrated against similar samples from the present day, for which temperature and other climatic variables are measured directly. What is learned is often limited, as are modern weather station records, to conditions at one region. However, measurements of CO2 and CH4 taken from isolated cores give a global picture, since these long-lived gases are uniformly distributed in the global atmosphere.
When combined, these various forms of paleo-data allow us to reconstruct an imperfect but ever-clearer picture of the climate of the past. All of them clearly indicate that climate varies, due to natural causes, on all time scales, from decades to millions of years.
A CAPSULE HISTORY OF CLIMATE CHANGE
A picture of what is known of climate changes through the last 100 million years is shown in terms of estimated surface temperature in Figures 1-6, and described briefly below.
The last 1000 years (Fig. 1)

Figure 1 Example of regional variations in surface air temperature for the last 1000 years, estimated from a variety of sources, including temperature-sensitive tree growth indices and written records of various kinds, largely from western Europe and eastern North America. Shown are changes in regional temperature in ° </°>C, from the baseline value for 1900. Compiled by R. S. Bradley and J. A. Eddy based on J. T. Houghton et al., Climate Change: The IPCC Assessment, Cambridge UniversityPress, Cambridge, 1990 and published in EarthQuest, vol 5, no 1, 1991.
A prominent feature found in some regions during the first centuries of the present millennium is a time of particularly mild temperatures, reaching maximum warmth in the 12th to 13th centuries. In some locations at that time, surface conditions may have been similar to today. However, it is not at all clear whether this climatic feature occurred at the same time in all places--an important distinction from the somewhat more uniform pattern in recent decades.
This so-called Medieval Warm Period was followed by a longer span of considerably colder climate, often termed the "Little Ice Age" (approximately 1450-1890), when the global mean temperature may have been 0.5- 1.0°C lower than today. At this time alpine glaciers moved into lower elevations, and rivers that rarely freeze today were often ice- covered in winter. Precipitation patterns also changed in many regions.
Several explanations have been proposed for climate oscillations of decadal-to-millennial scale. Changes in volcanism seem to play an important role in year-to-year climate variability, although there is less evidence that prolonged clusters of eruptions can cause cooling trends of decades or longer. Changes in the output of the Sun may also be important--as noted in an accompanying article in this issue--and solar-induced changes of decadal scale could alter the pace of projected warming during the next few decades. It is important to realize that future impacts of the Sun on otherwise- rising temperatures could be either negative or positive; for example, solar changes could also accelerate, for a time, an underlying warming trend.
Other explanations for decadal-to-millennial scale variations relate to the currently popular concept of "chaotic" interactions within the climate system. This process involves complex and inherently unpredictable interactions between, for example, the oceans and the atmosphere. Such interactions could cause the climate system to change or drift with time, resulting, quite by chance, in a period of cooling or warming that may persist for decades to centuries. The continued action of these non-deterministic effects might then restore conditions to something resembling the earlier state.
Some ocean-atmosphere interactions are more predictable. One example is the well-known El Niņo phenomenon: a surface warming of equatorial Pacific waters, persisting for a year or more, that has a widespread effect on climate and human affairs.
There are indications of decadal-scale warming trends in the Pacific that may bear some resemblance to the shorter and better-known, El Niņo phenomena. For example, one of these longer-term periods began in 1976-1977, and another may have started in 1988-1989. Although we lack a full understanding of the relative importance of these and other decadal fluctuations, there is hope that more can be learned in coming decades, in time to refine the accuracy of global warming projections.
An application of our understanding of ten to 100 year variability may be found in the present debate over several features of the temperature history of the last 100 years, and the fact that surface temperature has not consistently risen during this time at the rate predicted by simple greenhouse warming. The period from the 1950s through the early 1970s exhibited a cooling trend; and while global temperatures have been high over the last fifteen years, they have also been relatively stable.
Those who challenge greenhouse warming predictions point to such patterns as indications that the model predictions are seriously in error. However, most climatologists point out that natural oscillations of a decadal-scale can modify a greenhouse warming signal during the present, relatively early stage of the perturbation. This is but one example where an historical perspective can give insight into what at first appears as a troubling discrepancy between models and observations.
Were "natural" climatic variations of the sort that have characterized the last 1000 years to recur in the next 100 years or so, they could modify the expected effects of increased greenhouse gases: either masking an underlying upward trend during the early stages of a greenhouse warming or accelerating the rate at which it occurs. From what we know, however, the effect--either way--might not be great: only the extreme 1.0° C cooling estimate for the Little Ice Age approaches in magnitude the smallest temperature perturbation that is now projected for the end of the next century.
The last 15 thousand years(Fig. 2)

Figure 2: Variations in regional surface temperatures for the last 18,000 years, estimated from a variety of sources. Shown are changes in ° </°>C, from the value for 1900. Compiled by R. S. Bradley and J. A. Eddy based on J. T. Houghton et al., Climate Change: The IPCC Assessment, Cambridge University Press, Cambridge, 1990 and published in EarthQuest, vol 5, no 1, 1991.
The Medieval Warm Period and the Little Ice Age appear as minor, short-term fluctuations when we take a longer view. In Figure 2 we recognize these features as little more than ripples on the longer and more significant global warming of 4 to 5° C of the last 15 thousand years, that marks the recovery of the Earth from the grip of the last major glaciation, or Ice Age. The present warm epoch, beginning roughly 10 thousand years ago, is known geologically as the Holocene Interglacial. The "inter" is a reminder that such time intervals are relatively infrequent on time scales of a million years or so, and have lasted on average about 10 thousand years before a return to colder climates.
The onset and recovery from Ice Age conditions is now attributed to slow changes in the Earth's orbit--the so-called Milankovitch effect--that modify the seasonal cycle of solar radiation at the Earth's surface. These modifications bring about regional changes in both temperature and precipitation.
Especially affected in terms of rainfall are areas that fall under the influence of the African-Asian monsoon, which have experienced a long-term decrease in precipitation since the beginning of the Holocene, and particularly in Northern Africa and the Middle East. For example, several thousand years ago Neolithic or late Stone-Age man occupied the Tibesti Massif in what is now the driest part of the Sahara Desert. The development of agriculture in Mesopotamia and in the Indus Valley of present-day Pakistan and India benefited from the increased moisture that characterized the area during the end of the period of higher temperature: the so-called "Holocene Maximum."
Although regional temperatures during the Holocene Maximum were on the order of 1° C warmer than present, the warming did not occur at the same time in all places; thus the global average temperature may not have been significantly different from now. For this reason, we cannot turn to such times for reliable models, or analogs, of what is now anticipated, or cite them as evidence that humankind has in the past experienced increases in global temperature equivalent to those projected for even the early stages of enhanced greenhouse warming.
The last 150 thousand years (Fig. 3)

Figure 3 Air temperature near Antarctica for the last 150,000 years. Temperatures given are inferred from hydrogen/deuterium ratios measured in an ice core from the Antarctic Vostok station, with reference to the value for 1900. Compiled by R. S. Bradley and J. A. Eddy based on J. Jouzel et al., Nature vol 329, pp 403-408, 1987 and published in EarthQuest, vol 5, no 1, 1991.
Seen in the earlier portion of this more extended span of time is the onset and end of the last interglacial period, lasting about 10,000 years, as well as the much longer Ice Age that separated it from the present Holocene. The interglacial period that began about 130,000 years BP (before present) is often called the "Eemian." Regional temperatures were sometimes 1 to 2° C higher than those of the Holocene interglacial. However, there is less evidence that the temperature changes were globally synchronous, so in terms of global temperature change, conditions in the Eemian--once again--may not have been much different from the present.
At the time of maximum cold during the last Ice Age--about 15,000 to 23,000 years BP--ice sheets more than 2 kilometers, or well over a mile, in thickness, extended to about 40° latitude in North America, as far south as New York City and St. Louis. The massive amount of water in the ice sheets required evaporation of almost 50 million cubic kilometers of water from the oceans. As a result, sea level dropped about 105 meters, or about 350 feet, below present levels. The seas' retreat exposed most of the continental shelves, and allowed early man to migrate across the Bering Strait, eventually to populate all of North and South America.
The last Ice Age was also marked by an equatorward expansion of sea ice in both hemispheres. Ocean currents were displaced toward lower latitudes. Paleodata also suggest a significant reduction in the area of tropical rain forests, and an expansion of savanna vegetation that is typical of drier climates. There was also a nearly worldwide increase in the amount of dust in the atmosphere.
What is found in ice cores regarding CO2, CH4, and nitrous oxide (N2O) is particularly relevant to present concerns of anticipated greenhouse warming, for these are the same atmospheric trace gases that are now of such concern. We now know that each of them varied, systematically, with the coming and going of the Ice Ages (Fig. 4). During times of glacial cold their concentrations in the atmosphere dropped dramatically; during the Eemian and with the onset of the Holocene interglacial they rose to values typical of the air of pre-industrial times. The generally parallel behavior of surface temperature and atmospheric greenhouse gases supports the positive relationship between greenhouse gases and climate change that has long been discussed.
Figure 4: Concentrations of atmospheric greenhouse gases (in parts per billion by volume) over the last 50,000 years, compared to estimated changes in air temperature at high latitudes over the same period (bottom curve), derived from analyses of an ice core from Antarctica. After M. Leuenberger and U. Siegenthaler, Nature, vol 360, pp 449-451, 1992
The reasons behind some of the changes in trace gases during a glacial cycle are not fully understood, but it is likely that greenhouse gases have acted as a positive feedback to amplify smaller variations in surface temperature. For example, the amount of CO2 in the atmosphere probably varied in response to changes in ocean chemistry, for the ocean stores much more carbon than the land or the atmosphere. Changes in methane were most probably related to temperature-related changes in the global extent of wetlands, for wetlands (such as swamps and peat bogs) are today the primary source of this trace gas. Ice Age variations in N2O may have been tied to changes in marine biological activity, but the details of the process are as yet not well understood.
Since greenhouse gases appear to have played so important a role in modifying the climate of the last million years, one might ask to what degree "natural" changes in their concentrations could alter--or perhaps nullify--the projected impact of what we ourselves are adding to the atmosphere. To answer this question it is necessary to understand that the amount we have added to the atmosphere since the Industrial Revolution is already equal to the changes in concentration that accompanied the climatic variations of the Ice Ages. Since about 95 percent of the fossil fuel reservoir remains to be processed (see below), future increases are likely to overwhelm any naturally induced perturbations in the climate system.
The last million years (Fig. 5)
Figure 5 Estimate of surface temperature for the last 800,000 years, inferred from measurements of the ratio of 16O to 18O in fossil plankton that settled to the sea floor. The use of oxygen isotope ratios is based on the assumption that changes in global temperature approximately track changes in the global ice volume. Detailed studies for the last glacial maximum provide the temperature scale. Shown are changes in temperature in °</°>C from the modern value. Based on data from J. Imbrie, J. D. Hays, D.G. Martinson, A. McIntyre, A. C. Mix, J. J. Morley, N. G. Pisias, W. L. Prell, and N. J. Shackleton, in A. Berger, J. Imbrie, J. Hays, G. Kukla, and B. Saltzman, eds., Milankovitch and Climate, Dordrecht, Reidel, pp 269-305, 1984.
The jagged valleys in this reconstruction of temperature in the Pleistocene, or glacial epoch, are repeated Ice Ages. The warm peaks are interglacial periods--including the Eemian and at far right, the present Holocene. What is immediately obvious is how rare are times as warm as now. Ancestral Homo sapiens did not appear until the middle part of the period that is shown, and "modern" Homo sapiens (Cro-Magnon Man) not until the last 100,000 years.
The temperatures that are ascribed in this representation of the last 800,000 years were not obtained directly, but are based on fluctuations of global ice volume, and scaled to what is known of conditions during the last glacial maximum. They are meant to represent estimates of the mean surface temperature of the Earth.
As noted earlier, there is good evidence that seasonal changes in the way that sunlight is distributed--driven by periodic changes in the Earth's orbit--trigger both the waxing and waning of glacial ice. At the same time, climate model simulations suggest that these slow variations are not sufficient, in themselves, to account for all that is known of the glacial-interglacial fluctuations of the last million years. Resulting changes in greenhouse gas concentrations are now thought to amplify the effects of changes in solar radiation, through processes that are not entirely understood.
The last 100 million years (Figure 6)

Figure 6: A schematic reconstruction (solid line) of mean global surface temperature through the last 100 million years, based on analyses of various marine and terrestrial deposits. The dashed-line extension is a prediction of future trends through the coming 400 years, based on the assumption of substantial utilization of the fossil fuel reservoir. The vertical line shows the approximate range of surface temperature in climate model predictions for a doubling of CO2 levels, at about 100 years in the future. Modified from T. J. Crowley, Journal of Climate, vol 3, pp 1282-1292, 1990.
Paleodata of various kinds indicate that the Earth's climate prior to the last million years was considerably warmer. For almost all of the time from about 2 to at least 200 million years ago (Ma) the surface temperature exceeded that of today. The greatest warmth is found in what geologists call the Cretaceous Period, about 100 Ma, when the mean global surface temperature may have been as much as 6 to 8° C above that of today. This was followed by a fairly steady cooling, sometimes with abruptly stepped transitions, towards the unique glacial oscillations of the last few million years.
During most of this long period of time, and certainly from about 150 to 50 Ma, there is little evidence for ice sheets of continental scale, and subtropical plants and animals lived far poleward (almost 55-60° latitude) of their present limit of about 30°--the latitude of northern Florida. The Age of Dinosaurs, ending about 65 Ma, overlaps most of this warm interval, and fossilized remains of these large reptiles have been found on the North Slope of Alaska. Later, during the warmest part of the Age of Mammals (55 Ma), large trees grew in Arctic Canada (78°N), in regions that today are covered by tundra. Alligators and primates, also indicative of warm climate, have been found on nearby Ellesmere Island. Fossils of warm-water mollusks have also been recovered on the Antarctic Peninsula.
Climate model simulations suggest that large increases in CO2 are needed to explain the high temperatures of the Cretaceous. These results are in agreement with geochemical models that simulate the exchange of carbon and other elements among the ocean and air and land reservoirs, which imply that atmospheric CO2 levels have also varied on time scales of millions of years. There is growing evidence from the geologic record to support these conclusions. The geochemical models indicate that the concentration of this gas can be affected by long-term changes in several natural sources and reservoirs: CO2 emissions from volcanoes, the amount of carbon stored in the terrestrial biosphere, and the effect of water erosion of the land surface. The latter process affects the chemical weathering of surface rocks, altering their ability to remove CO2 from the atmosphere, and it can vary with changes in the amount of elevated terrain. The average height of each of the continents has changed over time, due to slow oscillations in the rate at which mountains are built; thus the weathering rates and the consequent effect on CO2 have also probably changed on time scales of millions of years.
Although the estimated levels of CO2 in the atmosphere for these very ancient times are uncertain to within a factor of three to four, it is interesting to note that the amount thought to be present in the air of the Cretaceous is comparable to estimates of what now remains in the fossil fuel reservoir: the yet unused amount of coal and the hydrocarbon fuels that include crude oil, natural gas, oil shales, and oil sands. Coal is far and away the major constituent, and it will supply most of the carbon for future increases in greenhouse gases. Coal is about ten times more plentiful than all the sources of hydrocarbon, and about thirty times more abundant than crude oil in particular. Utilization of all known or likely reserves of crude oil would add only about 25 percent to the carbon dioxide now present in the atmosphere. For reference, about 5 percent of the available fossil fuel reservoir has thus far been utilized, and a doubling of CO2 levels will consume only about 20 percent.
Barring a radical change in the manner in which energy is utilized in the future, continued depletion of the fossil fuel reservoir in the next few centuries could result in levels of atmospheric greenhouse gases that are comparable to the warm time period of the Cretaceous. The warming that is calculated to result from nearly full utilization of the fossil fuel reservoir is also consistent with independent estimates of temperatures in the Cretaceous. Thus the geologic record yields the rather startling conclusion that the climate of AD 2400-2700 could be comparable to that experienced during the Age of Dinosaurs, which was as warm as any time in the last billion years.
LESSONS FROM THE PAST
As we have seen, the geologic record leaves no doubt that the Earth's climate system is capable of some very large changes. This almost trivial conclusion challenges any presumption that the surface temperature will, through natural checks and balances, remain stable in the face of perturbations that we now impose. The geologic record also provides insight into specific characteristics of the climate system that relate to future greenhouse warming. Some of these are outlined below.
Times of similar temperature
Of obvious interest are comparisons of the surface temperatures now foreseen by climate models with what the Earth has experienced in the past. The projected warming of several degrees C has occasionally prompted comparisons with the last, or Eemian, interglacial period of about 125,000 years ago (Fig. 3), when regional temperatures were at times and places sometimes warmer than the present. As noted earlier, the qualification is important, for further study has revealed that these times of warming pertain only to specific regions, and not to a simultaneous, worldwide change. As an analog, the Eemian is thus of little help.
To find times when global-averaged surface temperatures significantly exceeded those of the present day--reaching, for example, the 2.0-2.5°C mid-range of IPCC projections--we need look several million years into the past (Fig. 6). To evaluate the full potential of future greenhouse gas increases, including what will ensue when much of the available fossil fuel reservoir has been consumed, we must look much further back in time.
Assessments of fossil fuel use and deforestation suggest that concentrations of atmospheric CO2 could eventually increase to six or seven times the pre-industrial level. Were severe conservation methods imposed, greenhouse gases would still rise to about two and a half times the present concentrations--or slightly more than three times the pre-industrial level--within the next few centuries. Such concentrations would likely raise global temperatures to a range that has not been experienced since the early part of the Age of Mammals, about 55 million years ago.
Still, when major climatic changes are involved, it is hazardous to take any period in the distant past as a reliable analog for how the climate system will respond in the future. One reason is the difference in geography: the continents drift with time, and during the Cretaceous their placement was quite different from today, as were the contours of mountains and other features of surface relief. In addition, the very rapid change in surface temperature that is now projected will result in unstable or non-equilibrium climates. The reason is that neither the deep oceans nor ice sheets--which can respond only slowly--will be able to keep pace with changes in air temperature.
One implication of the rapid changes that are projected is that the Greenland and Antarctic ice caps will persist in the presence of global air temperatures that are normally associated with an ice-free Earth. I know of no time period in Earth history with a combination of very high CO2 levels, polar ice caps, and a non-equilibrium climate.
Potential for abrupt transitions
It has long been thought that the great Ice Ages came and went on time scales measured in thousands of years, and less momentous changes--such as the Holocene Maximum or the Little Ice Age--over the span of several centuries. Current studies and more recent paleodata have revealed quite another face of the climate system, called "abrupt transitions," in which major shifts in some components of the Earth's climate are accomplished on time scales of decades or less.
Initially proposed, and later verified, was the revolutionary notion that the large-scale circulation in the North Atlantic could persist in one of two patterns, or states, both of which were quite stable, with the possibility of abrupt switching between the two. In the first, the warm Gulf Stream that flows along the eastern coast of the U.S. continues northward, reaching beyond the British Isles to the Norwegian Sea, ameliorating the climate of northwest Europe. James Joyce aptly referred to this condition in Ulysses, when he wrote that "All Ireland is washed by the Gulf Stream."
In the other possible mode, the northward extension of the Gulf Stream is weakened by a reduction in the salinity of surface waters in high latitude regions of the North Atlantic. With less salt, seawater is not as dense, and is less able to sink during normal wintertime cooling. Restricting the ability of the North Atlantic to circulate water downward limits the amount flowing in from the warm Gulf Stream. The result of this "short-circuit" in ocean circulation is a much cooler climate for all who live downstream, including Northern Europe.
The surprising evidence from the paleoclimate record is how quickly the switch between warm and cold states can be accomplished. Evidence from ice-age portions of recent Greenland ice cores suggests that changes of this sort may have taken place in the past in the span of five to ten years. These abrupt transitions are most likely linked to an increase in the release of icebergs from continental glaciers, which on melting contribute large volumes of freshwater into the ocean, systematically reducing the local salinity.
Whatever the cause, we now know that in at least the North Atlantic the climate system can change very rapidly. Might ocean circulation change as rapidly in the future, perhaps as a consequence of other significant changes in the system? The answer is "maybe." There are no permanent ice sheets today on the North American continent, as was the case in the past, but melting of Arctic sea ice or the extensive Greenland ice cap could well influence ocean salinities. Increased precipitation over the North Atlantic, induced by warmer temperatures, could also reduce the saltiness of seawater, short- circuiting the ocean circulation in a manner similar to what occurred during the ice ages. In fact, greenhouse models call for such a change in precipitation, and the present rate of warming in the subpolar North Atlantic--less than what is recorded in the rest of the world--is also in agreement with what should happen as a result of an altered state of ocean circulation. A test of the models is whether the slower warming of the subpolar North Atlantic will persist.
Checking climate model results against paleodata
Even if there were no period in the past that could serve as an analog for future climate, the geologic record can still provide valuable insight into the modeling of specific processes in the climate system. Examples of such processes include the response of climate to changes in freshwater input to the ocean, or to changes in the contour of the land. These changes have occurred in the past and have left their traces in the geologic record. Incorporating more accurate predictions of specific processes will improve the overall reliability of global climate models.
Testing general circulation models against what is known of past climatic changes provides powerful insights, particularly when applied to regional, as opposed to global, changes. In many cases these reality checks have bolstered our confidence in numerical simulations of the atmosphere and oceans and other parts of the climate system. Some examples are the temporal evolution of the African-Asian monsoon; the wet conditions in the U.S. Southwest during the last Ice Age; the expansion of Antarctic sea ice during the last glacial maximum; changes in deep water circulation both during the Pleistocene and on longer time scales; regional climate trends due to changes in mountain heights over the last few million years; formation and fluctuations of glaciers during the ice ages at 300 and 440 million years ago; and the formation of coal deposits that are now found in the eastern U.S. These and other results have been obtained with the same climate models that are now used to project impending greenhouse warming.
Surface temperatures derived from paleodata reveal that when the global temperature warms, changes in polar regions are systematically larger than nearer the equator. Climate models predict a similar distinction between polar and lower latitude regions with future warming, but with differences that are less than what have been found in available data from the past. It may be that this discrepancy reflects an inherent limitation in the use of paleodata for precise validation of climate models. Or, the models may not adequately simulate the manner in which ocean circulation transports heat from the equator to the poles. If this is so, some of the regional predictions of climate models may be in error in regions strongly influenced by ocean heat transport.
We can better understand the differing degrees of success with which models replicate climate changes of the past by distinguishing between global and regional temperatures. In general, climate models do a better job of estimating changes in global temperatures, because the energy budget of the entire planet is affected. In contrast, regional changes are a step removed: they reflect the response of the atmosphere and ocean circulation to changes in the total energy budget, and as a result, are more difficult to predict. In some cases the models have still yielded valid first-order predictions of what is found in paleo records. In others, the modeled projections do not agree as well. Some of these discrepancies may tell not so much about model deficiencies as about our inability to read what Nature has written in the record of the past.
SUMMARY AND CONCLUSIONS
The paleo record provides a wealth of information relevant to current concerns of enhanced greenhouse warming, including the underlying truth that the Earth's climate has experienced substantial changes in the past.
Major, known changes of surface temperature in the past correlate well with variations in atmospheric greenhouse gases and appear to be caused or amplified by them. The mid-range scenarios employed in IPCC projections for the end of the next century would result in global mean surface temperatures that exceed any well-documented warming in the last million years. The mid- to high-end "out-century" estimates for temperatures are as high as any known for the last one billion years. The combination of high air temperatures, polar ice caps, and non-equilibrium climate defines a climatic condition that may be unprecedented in Earth history. Paleodata also support earlier suggestions that the subpolar North Atlantic region may be susceptible to abrupt climatic transitions as a result of changes in the salinity of surface waters.
The sulfate aerosols that are also added to the atmosphere when fossil fuels are burned act to cool the climate, and the degree to which these emissions might reduce the impact of greenhouse gases is now under intensive study. Could such effects ameliorate some of the more extreme projections that are discussed above?
While this is indeed possible we should not forget that other possible mechanisms could act in the opposite direction, to amplify climate change. Among such "positive feedbacks" are significant increases in atmospheric concentrations of trace gases other than carbon dioxide, such as methane, nitrous oxides, and chlorofluorocarbons. In fact, recent IPCC estimates suggest that the global climate forcing from the other greenhouse gases is comparable in magnitude, but approximately opposite in sign, to that of sulfate aerosols.
Global warming could very well accelerate the release of carbon from soils to the atmosphere. Possible changes in the ocean circulation and ocean productivity could also increase the amount of carbon that enters the atmosphere. Both positive and negative feedbacks therefore need to be considered in evaluating the uncertainty of current climate projections.
The conclusions given above, despite uncertainties, represent in my opinion a reasonable assessment of the significance of the greenhouse perturbation when viewed from the perspective of the geologic record. The net impression of this evaluation of "things past" is that the future climate promises to look very different than the present and, perhaps more disconcertingly, possibly unlike anything known before.
FOR FURTHER READING
"Atmospheric carbon dioxide over Phanerozoic time," by R. A. Berner. Science, vol. 249, pp 1382-1386, 1990.
Climate Change: The IPCC Scientific Assessment, Edited by J. T. Houghton, G. J. Jenkins, and J. J. Ephraums. Cambridge University Press, 364 pp, 1990.
"Global climatic change," by R. A. Houghton and G. M. Woodwell, Scientific American, vol. 260, no. 4, pp 36-44, 1989.
"How sensitive is the world's climate?" by J. Hansen, A. Lacis, R. Ruedy, M. Sata, and H. Wilson. National Geographic Research and Exploration, vol. 9, pp 143-158, 1993.
Paleoclimatology by T. J. Crowley and G. R. North. New York, Oxford University Press, New York, 339 pp, 1991.
"Unpleasant surprises in the greenhouse?" by W. S. Broecker. Nature, vol. 328, pp 123-126, 1987.
Reviewers
Anthony Broccoli is a meteorologist and climate modeler at the Geophysical Fluid Dynamics Laboratory of the National Oceanic and Atmospheric Administration, located at Princeton University in New Jersey. He is interested in the study of climate change using numerical models, particularly the simulation of past climates.
Dr. William Ruddiman is a marine geologist and currently chair of the interdisciplinary Department of Earth Sciences at the University of Virginia in Charlottesville. His main research interests are the generation and interpretation of paleodata from many periods of Earth history, with particular emphasis on the effects of plateau uplift on climate.
Dr. Lisa C. Sloan is a geologist and paleoclimate modeler, and an assistant professor in Earth Sciences at the University of California, Santa Cruz. Her primary interests are in the modeling of warm intervals of Earth history and in comparisons of geologic data with climate model results.
Scientific reviewers provide technical advice to the authors and Editor, who bear ultimate responsibility for the accuracy and balance of any opinions that are expressed.
Back to Climate Change Page Back to English Version
See the weather in Argentina

You are visitor No.:

since January, 2002
FastCounter by bCentral
See here many interesting
statistics about this site
Which countries see us?
Who are our visitors?
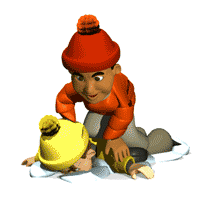
Don't get angry!
Just tell us your opinion!